LEAP - Long-range Energy Alternatives Planning System
Short overview
LEAP, the Long-range Energy Alternatives Planning System, is a widely-used scenario-based software tool, which supports a wide range of different modelling methodologies for energy policy analysis and climate change mitigation assessment at many different scales, ranging from cities and states to national, regional and global applications. On the demand side, these applications range from bottom-up end-use accounting techniques to top-down macroeconomic modelling. LEAP’s functionalities lay within the core activities of countries undertaking integrated resource planning, greenhouse gas (GHG) mitigation assessments, and Low Emission Development Strategies (LEDS), especially in the developing world. The main purpose of LEAP lies in providing a means of comparison between a “Business as Usual (BaU)” scenario, and other user-customised scenarios promoting the adoption of innovative policies and sustainable strategies in the context of a country, in crucial activity sectors, such as the energy production and the building envelope. LEAP supports numerous scenarios, thus providing for in-depth insights regarding a wide variety of key energy, environmental and socioeconomic indicators. At least 32 countries used LEAP for a variety of tasks focused on the field of GHG mitigation by several techniques, such as changing the energy consumption on the demand side, shifting the energy carrier on the supply side and imposing policies on the supply and demand sides (Martı́nez-Jaramillo et al., 2017) in the context of creating energy and emissions scenarios, to be the basis for their Intended Nationally Determined Contributions (INDCs) to climate action. (Dagher and Ruble, 2011) constructed LEAP-based scenarios and examined the technical, economic, and environmental implications of all scenarios with regard to electrical planning. Using the LEAP model, Jun and Lee (2010) focused on the penetration of renewable energies on the existing electricity generation market in South Korea, in terms of economic and environmental influence. (Cai et al., 2007) assessed the reduction potential of CO2 emissions in China’s electricity sector and simulated different development paths.
LEAP is based on an integrated, scenario-based structure, which can also be used to track energy consumption, production and resource extraction in all sectors of an economy, including accounting for GHG emission sources and sinks at both the energy and non-energy sectors. LEAP’s structure enhances the representation of the energy system of each Member State by breaking down the energy used in each economic activity, even to the “lowest” level (i.e. households). It also allows for the modelling of several energy production units (e.g. lignite, natural gas, renewables, etc.) along with their technical specifications (e.g. power, technical minimum, lifetime, fuel, etc.) as well as optimised unit commitment, in terms of meeting the end-user demand curve with the minimum cost. Energy CO2 emissions and other greenhouse gases (e.g. CO2 from others sources, CH4, N2O, HFCs, PFCs and SF6) are then calculated by the model, with respect to the energy production side. In addition to tracking GHG emissions, LEAP can also be used to analyse emissions of local and regional air pollutants, and short-lived climate forcers (SLCFs), making it well-suited to studies of the climate co-benefits of local air pollution reduction. Given LEAP’s diversity, it can be used to explore GHG mitigation alternatives through energy system transformations as well as to provide policy insights towards transitioning to a climate-resilient energy sector.
Key features of the LEAP tool
Energy sectoral detail
On the demand side, each sector is modelled as a production or an energy intensity level and the use of other key intermediate factors (capital, intermediate consumptions, etc.) given its expectations on demands and prices. Regarding the end-user side specifically, the final demand is usually broken down into four major categories: a) residential, b) agricultural, c) commercial and d) transport, providing the user with the capacity to introduce all associated information, such as (non-) electrified households, passenger- and ton-km, etc. As we move further from the demand side, LEAP allows the user to model the energy production side, along with their respective networks and implications (e.g. resource limitations and energy losses). This approach can be used to fully describe individual policy measures which can then be combined in different combinations and permutations into alternative integrated scenarios, thus allowing policy makers to assess the impact of an individual policy as well as the interactions that occur when multiple policies and measures are combined, in terms of both energy and environmental impact.
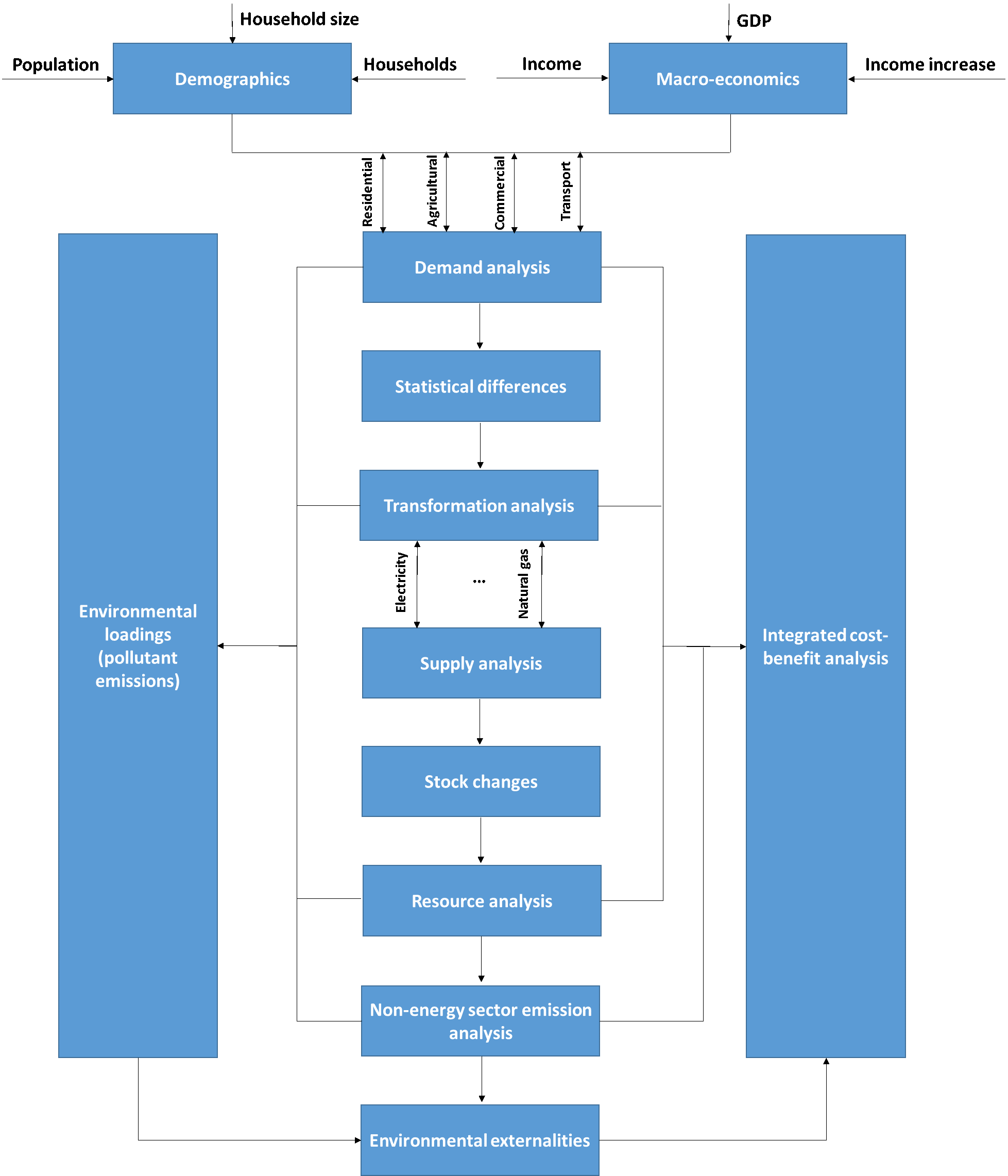
Representation of the LEAP energy system Source: LEAP User Guide, 2005; own elaboration
Geographic coverage
LEAP provides a range of accounting, simulation and optimisation methodologies that are powerful enough to easily incorporate data and results from other more specialised models, thus making LEAP an integrated tool spanning across the entire globe, at national, regional and local level (see the table below).
Geographic region | Countries |
---|---|
Africa | Algeria, Angola, Benin, Botswana, Cameroon, Congo, Democratic Republic of Congo, Côte d’Ivoire, Egypt, Eritrea, Ethiopia, Gabon, Ghana, Kenya, Libya, Morocco, Mozambique, Namibia, Nigeria, Senegal, South Africa, Sudan, United Republic of Tanzania, Togo, Tunisia, Zambia, Zimbabwe, and Other Africa |
Australia, New Zealand | Australia, New Zealand |
Canada | Canada |
North America | US, Mexico |
Latin America | Argentina, Bolivia, Brazil, Chile, Colombia, Costa Rica, Cuba, Dominican Republic, Ecuador, El Salvador, Guatemala, Haiti, Honduras, Jamaica, Netherlands Antilles, Nicaragua, Panama, Paraguay, Peru, Trinidad and Tobago, Uruguay, Venezuela and Other Latin America |
Central and Eastern Europe | Albania, Bosnia-Herzegovina, Bulgaria, Croatia, Czech Republic, Hungary, Macedonia, Poland, Romania, Serbia and Montenegro, Slovenia, Slovakia |
Middle East | Bahrain, Islamic Republic of Iran, Iraq, Israel, Jordan, Kuwait, Lebanon, Oman, Qatar, Saudi Arabia, Syria, United Arab Emirates, Yemen, and Turkey, Cyprus |
Russia and Central Asia | Armenia, Azerbaijan, Belarus, Estonia, Georgia, Kazakhstan, Kyrgyzstan, Latvia, Lithuania, Moldova, Tajikistan, Turkmenistan, Ukraine, Uzbekistan, Russian Federation |
(Other) South and Southeast Asia | Bangladesh, India, Brunei Darussalam, Cambodia, Taiwan (China), Indonesia, DPR of Korea, Malaysia, Mongolia, Myanmar, Nepal, Pakistan, Philippines, Singapore, Sri Lanka, Thailand, Vietnam and Other Asia (Japan, China, Republic of Korea, etc.) |
Western Europe | Austria, Belgium, Cyprus, Denmark, Finland, France, Germany, Greece, Iceland, Ireland, Italy, Luxembourg, Malta, Norway, Portugal, Spain, Sweden, Switzerland, The Netherlands, UK |
Multi-year time periods
LEAP is intended as a medium- to long-term modelling tool. Its calculations occur based on a user-defined time-step (e.g. annual, 5-year period, etc.) and baseline year (e.g. 2005, 2010, etc.), and the time horizon can extend to an unlimited number of years (beyond 2100). Studies typically include both a historical period known as the Current Accounts, in which the model is run to test its ability to replicate known statistical data, as well as multiple forward-looking scenarios. Typically, most studies use a forecast period of between 20 and 50 years.
Intra-year time periods (time slices)
Some results are calculated with a finer level of temporal detail. For example, the year can be split into different user-defined “time slices” to represent seasons, types of days or even representative times of the day. These slices can be used to examine how loads vary within the year and how electric power plants are dispatched differently in different seasons. LEAP includes an easily-customised dataset of time-slices, which extends from seasonal segregation (i.e. summer, winter, autumn, fall) today- and night- time of all seasons as well as hourly representation. This kind of in-depth time-slicing seeks to provide analytical insights and partially offset the stochastic nature of the energy production sector (given the high RES penetration) and the fluctuating end-user demand across the year, by facilitating the matching of supply and demand in each time-slice.
Climate module & emissions granularity
The climate component in LEAP includes a diverse database of both energy and non-energy sector GHG emissions per fuel or activity, thus calculating the final GHG emissions stemming from an energy system’s operation at global, national, regional, or local level, based on the initial energy and socioeconomic data provided (e.g. demographics, income, GDP, etc.). The tool tracks far beyond the three main sources of greenhouse gases (i.e. CO2, CH4, and N2O), extending its analysis to tracking SLCFs such as fluorinated gases, black carbon, etc. The LEAP model also includes the Technology and Environmental Database (TED), which provides extensive information describing the technical characteristics, costs and environmental impacts of a wide range of energy technologies (including existing technologies, current best practices and next generation devices), compiled from numerous sources (e.g. Eurostat, EEA, etc.). Thus, LEAP is appropriate for energy policy analysis and climate change mitigation assessment. Since LEAP is a scenario-based modelling tool, its climate module calculates changes in the atmospheric concentration of all implicated GHG emissions between a reference scenario (which depicts the current condition of a system in terms of energy—demand and supply—and demographics—population, income, etc.) and a number of GHG mitigation-oriented scenarios based on current and future limitations, aligned with the ongoing global treaties and agreements (such as the goals set by the Paris Agreement or by increasing ambition).
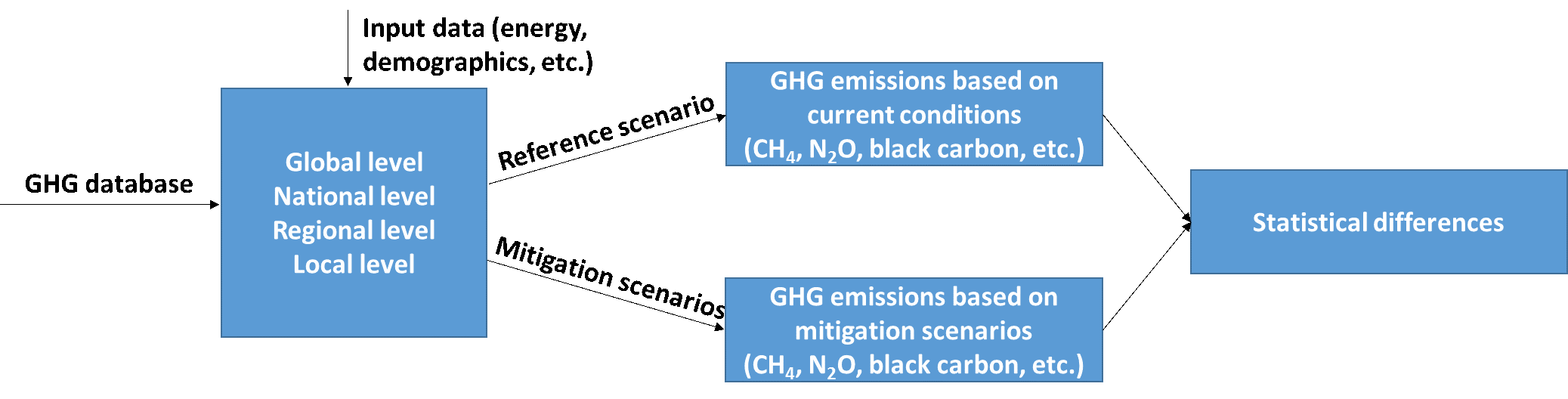
Logic of LEAP climate module Source: LEAP User Guide, 2005; own elaboration
Socioeconomic dimensions
LEAP requires in-depth inputs regarding the changes in socioeconomic conditions (e.g. population, household size, etc.) and energy sector (e.g. supply and demand), as well as demand for other goods and services that affect GHG emissions (such as agricultural demand) for both the reference and the mitigation scenarios, over the time horizon of the analysis. It should be noted that LEAP does not include a default set of socioeconomic and energy data, since it is an easily-customised tool that can be adjusted to use several socioeconomic and sectoral growth projections from different sources (aside from SSPs). Since the economic, population and sectoral growths are used to capture specific drivers for the changes in energy demand in a reference scenario (in which no climate change mitigation measure has been applied), LEAP allows users to self-assess and define the sets of energy service demands over the horizon, as well as to quantify them based on a diverse set of quantification units. This set of quantification varies from passenger-kms and tonne-kms in the transport sector to kWhs and metric tonnes in the industrial sector, as well as to Gigajoules of final consumption per unit of activity level (GJ/€). LEAP is also widely open to conducting cost-benefit analyses if the required socioeconomic and energy inputs needed are partially available.
Calibration of the model
LEAP is calibrated for the initial period, in terms of fuels, based on a standard fuels database drawing data from IEA, UN and other standard international sources (such as the IPCC). These data sources should suffice for most LEAP analyses. However, LEAP allows the user to edit the default data, for example to change the energy contents of certain fuels (such as coal and wood) to reflect the conditions in the area they aim to study. In addition, LEAP provides an “Add” and “Delete” button for the user to add a new fuel and delete one respectively, if necessary, and manually define its respective specifications. Regarding the projections for energy in the reference and mitigation scenarios, the installed technological capacity along with its CAPEX and OPEX, the exported, imported, produced and consumed quantities for all energy carriers etc., LEAP does not include a respective database, and therefore this kind of data must be entered and updated manually.
Mitigation/adaptation measures and technologies
LEAP includes a wide dataset of the most major fossil fuel and low-carbon technologies that are perceived to be commonly exploited in the upcoming years. Thus, for each economic activity, the energy demand is broken down in key categories such as oil, gas, solid fuel, biomass, etc. Regarding the electricity sector, LEAP allows for an in-depth representation of the energy mix capability by providing several energy-based production units such as, solar, nuclear, wind, etc. By simulating the substitution of low-carbon for high-carbon technologies in response to their relative costs, as well as emissions constraints, the LEAP model simulates mitigation. The principal energy sector CO2 mitigation technology options are as shown in the following table. The plethora of energy choices that LEAP provides can be crucial for the suggestion of several mitigation/adaptation policies. These scenarios may aim to the reduction of GHG emissions or the abatement of the cost of energy production. An interesting example is the choice between hydroelectric power and nuclear power. Both energy sources are emission-free but each of them features different costs depending on the region they are applied.
Electricity and heat | |
---|---|
Electricity generation | Heat generation |
|
|
Transport |
|
Road | Rail |
|
|
Air | Marine |
|
|
Buildings |
|
Heating | Lighting |
|
Efficiency |
Appliances | Cooling |
Efficiency |
|
Industry |
|
Process heat | Steam |
|
|
CHP |
|
|
|
Agriculture |
|
Energy use |
|
|
Economic rationale and model solution
LEAP simultaneously calculates the quantity of the energy produced, converted and consumed with regard to the different “commodities” accounted for in the model, the total GHG emissions stemming from a system’s operation, as well as the way in which these emissions are broken down into each sector, in a given region or economy under a range of alternative assumptions on population, economic development, technology, prices and so on. These commodities comprise the different energy forms, the deployed technologies, and the energy services. LEAP allows to reach a cost-minimising level of commodity production and consumption, consistent with meeting all current and future energy demands and emissions constraints. The total energy system cost is calculated as a Net Present Value (NPV) cost of the energy system over the entire pre-defined time horizon. With its flexible data structures, LEAP allows for analysis as rich—in technological specification and end-use detail—as the user chooses. All of the aforementioned features contribute to the creation and evaluation of multiple alternative mitigation scenarios. These scenarios can be compared with a reference scenario (which projects the progress of energy demand, energy production, social costs and GHGs emissions of the region studied if no significant changes occur on the current energy system), in order to evaluate the contingent reduction of GHG emissions or social costs of the different mitigation scenarios suggested.
Policy questions and SDGs
Key policies that can be addressed
LEAP can be used to project the energy supply and demand situation in order to get a glimpse of future patterns, identify potential problems, and assess the likely impacts of energy policies. In this respect, LEAP allows to examine a wide variety of projects, programs, technologies and other energy initiatives, and arrive at strategies that best address environmental and energy problems. Some indicative policies that can be implemented in LEAP are the following:
- Minimum/maximum capacity factors on fossil fuel power generation plants (e.g. to simulate minimum or maximum desired levels of operation);
- Constraints on the CO2-related primary energy resources exploited (e.g. limit the availability of fossil fuels down to its minimum in the upcoming years);
- Energy consumption cuts through increasing energy efficiency on the end-users’ side (e.g. withdrawal of an outdated car fleet and replacement with new vehicles, based on cleaner fuels with lower consumption, such as CNG);
- Subsidies on particular technologies (through adjusting their costs);
- Constraints on the availability of particular technologies (e.g. “no nuclear”);
- Constraints on the growth rates of particular technologies (e.g. natural gas units and substitution with renewable energy sources);
- Constraints on the growth rates of particular socioeconomic aspects (e.g. population, income, GDP, etc.);
This allows LEAP to perform a number of policy-relevant investigations, such as:
-
What impact will current efforts towards expanding clean energy resources have
over time and which policies are the most effective, in terms of both minimising
costs and maximising social benefits?
- For example, Abt Associates (2017) explore the costs and benefits stemming from the implementation of different mitigation options both in the energy and transport sectors of Azerbaijan, Kazakhstan, and Uzbekistan, in terms of GHG abatement and social benefits. The effectiveness of these options was assessed in terms of GHG abatement, and social benefits.
-
Where can we do more to promote a green future?
- For example, Ouedraogo (2017) and Emodi et al., (2017) showcase the mitigation potential in different activity sectors in Africa (through the analysis of several mitigation scenarios), and their close association with socioeconomic variables such as gross domestic product, income per capita.
-
What amount of clean energy is needed to adequately reduce carbon pollution and
meet current emissions targets?
- For example, Mirjat et al., (2015) explore the energy supply mix that needs to be produced in order to meet Pakistan’s final energy demand, in alignment with the national and global GHG emissions reduction target.
Implications on other SDGs
LEAP does not automatically calculate the implications on non-climate SDGs of its least-cost energy system to meet prescribed climate or emissions constraints. However, it is possible to exploit LEAP’s outputs and conduct “off-model” calculations to estimate many of the SDG implications. For example, LEAP reports the total final energy consumption in an energy system. This energy can be reshaped at lower/higher levels, depending on the successful implementation of different mitigation scenarios, thus allowing for an estimation of the energy-poor citizens.
Recent publications using the LEAP model
Paper | Topic | Key findings |
---|---|---|
Mirjat et al., (2015) | Long-Term Electricity Demand Forecast and Supply Side Scenarios for Pakistan (2015-2050): A LEAP Model Application for Policy Analysis. | Given that the balance between demand and supply of electricity in Pakistan is yet to be achieved, in this study, Long-range Energy Alternatives Planning System (LEAP) is used to develop Pakistan's LEAP modelling framework for the period 2015–2050, through four supply side scenarios, following the demand forecast. In the most effective scenario (Energy Efficiency and Conservation) there is a CO2 reduction higher than 50% on the in 2050. |
Emodi et al., (2017) | Energy policy for low carbon development in Nigeria: A LEAP model application. | This paper applied a scenario-based analysis to explore Nigeria's future energy demand, supply and associated GHG emissions from 2010 to 2040 through the analysis of a reference scenario, a low-carbon moderate scenario, a low-carbon advanced scenario, and a green optimistic scenario. It is observed that in the Green Optimistic scenario the emissions will be 11% lower than in the reference scenario. |
Martı́nez-Jaramillo et al., (2017) | Assessing the impacts of transport policies through energy system simulation: The case of the Medellin Metropolitan Area, Colombia. | This paper quantifies the emissions that could be avoided by the implementation of Medellin’s Master Plan, the promotion of telecommuting, and the development of a transport energy model for the Medellin metropolitan area between 2010 and 2040. The results indicate that a policy combining the promotion of mass transportation could represent 5.65 Million Tons of CO2 equivalent avoided by 2040 (a 9.4% reduction). |
Abt Associates (2017) | Economics of Climate Change in Central and West Asia. | LEAP was used as the main modelling tool for analysing the costs and benefits of mitigation options in the energy and transport sectors of Azerbaijan, Kazakhstan, and Uzbekistan. The effectiveness of the examined options was assessed in terms of GHG abatement, and net social costs and benefits. |
Ouedraogo (2017) | Energy futures modelling for African countries. | An assessment of the current and future trends in energy demand in Africa and associated GHG emissions. Future energy demand is forecasted on the basis of socioeconomic variables such as gross domestic product, income per capita, population, and urbanisation. LEAP is applied to analyse and project energy demand and the related emissions under alternative strategies for the period 2010–2040. |
Huang et al., (2011) | The long-term forecast of Taiwan’s energy supply and demand: LEAP model application. | The Taiwan LEAP model is used to compare future energy demand and supply patterns, as well as greenhouse gas emissions, for several alternative scenarios of energy policy and energy sector evolution. An interesting conclusion of this study is the fact that if the existent nuclear plants are retired but not replaced by RES, there will be a higher demand for coal and other fossil fuels, which will result in the increase of greenhouse gases emissions. |
References
Martı́nez-Jaramillo, J. E., Arango-Aramburo, S., Álvarez-Uribe, K. C., & Jaramillo-Álvarez, P. (2017). Assessing the impacts of transport policies through energy system simulation: The case of the Medellin Metropolitan Area, Colombia. Energy Policy, 101, 101-108. doi:10.1016/j.enpol.2016.11.026
Cai, W., Wang, C., Wang, K., Zhang, Y., & Chen, J. (2007). Scenario analysis on CO2 emissions reduction potential in Chinas electricity sector. Energy Policy, 35, 6445-6456. doi:10.1016/j.enpol.2007.08.026
Ouedraogo, N. (2017). Energy futures modelling for African countries: LEAP model application. WIDER Working Paper, United Nations University - UNU-WIDER.
Emodi, N. V., Emodi, C. C., Murthy, G. P., & Emodi, A. S. (2017). Energy policy for low carbon development in Nigeria: A LEAP model application. Renewable and Sustainable Energy Reviews, 68, 247-261. doi:10.1016/j.rser.2016.09.118
Mirjat, N., Uqaili, M., Harijan, K., & Valasai, G. (2015). Pakistan’s Energy System: Integrated Energy Modelling and Formulation of National Energy Policies. International Conference on Sustainable Energy Technologies – SET2015, Nottingham, vol. 14.
Abt Associates. (2017). Economics of Climate Change Mitigation in Central and West Asia. Asian Development Bank. Asian Development Bank. doi:10.22617/rpt178634
Huang, Y., Bor, Y. J., & Peng, C.-Y. (2011). The long-term forecast of Taiwan's energy supply and demand: LEAP model application. Energy Policy, 39, 6790-6803. doi:10.1016/j.enpol.2010.10.023